Red Blood Cell Count (Low & High)
Red blood cells (RBCs) are cells that circulate in the
blood that specialize in delivering oxygen to the
body’s tissues. Because of the critical life-sustaining
function that oxygen has for the human body, they
are the most common type of blood cell (about 25%
of all cells in the human body and 40 to 45% of cells
in the blood). Red blood cells perform this important
role for humans and are the main way that all animals
with a backbone (vertebrates) get oxygen delivered
to the tissues.
Close up: Red blood cells.
The red blood cells take in oxygen from the lungs (or gills) and release it through the cell
membranes when they squeeze through the capillaries (where the oxygen level is low) to
tissues throughout the body. Capillaries are tiny blood vessels that act as an exchange
system connecting the smallest veins with the smallest arteries. Veins carry blood to the
heart whereas arteries carry blood away from the heart and to the tissues. In the
capillaries, oxygen is released and used by the cells of the body. It takes red blood cells
about 20 seconds to complete one cycle of circulation in humans.
"Where Medical Information is Easy to Understand"™
WHAT IS THE STRUCTURE AND SHAPE OF
RED BLOOD CELLS LIKE?
cell volume). Cytoplasm is a gel- like substance
that fills up a cell. Hemoglobin is a complex protein
that makes up the majority of the content of red
blood cells. Hemoglobin contains iron which
temporarily binds to oxygen and helps carry 97 to
98% of it to the lungs (or gills) and releases it to
cells throughout the body.
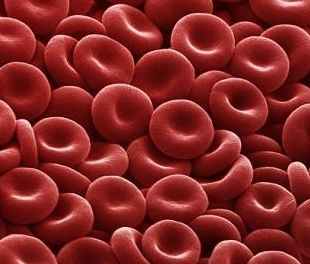
The remaining oxygen is carried in the blood plasma in dissolved form. Plasma is the fluid
component of the circulating blood that is watery and straw-colored. Hemoglobin allows
the blood to transport 30 to 100 times more oxygen than can be dissolved in the plasma
alone. Each hemoglobin molecule has four iron atoms, each of which can bind to a
molecule of oxygen. Each oxygen molecule has two oxygen atoms (known as O2).
This means that for each hemoglobin molecule there will be four oxygen molecules or eight oxygen atoms.
After hemoglobin releases oxygen, it binds to the tissue’s carbon dioxide (a gaseous waste product) or
other waste gases. Hemoglobin also carries some of the carbon dioxide back from the tissues. It is the
iron inside hemoglobin that helps transport oxygen and carbon dioxide. Ultimately, red blood cells carry
carbon dioxide to the lungs where it is eliminated during breathing. Red blood cells contain an enzyme
called carbonic anhydrase, which helps the reaction of carbon dioxide and water to occur 5,000 times
faster. When carbon dioxide and water combine, it is known as carbonic acid. This then separate into
hydrogen ions and bicarbonate (carbon, hydrogen, and three parts of oxygen). The hydrogen ions then
combine with hemoglobin and the bicarbonate ions go to the plasma. This is how about 70% of the body’s
oxygen is removed. About 23% of the carbon dioxide combines with hemoglobin and is released into the
lungs. The other 7% is dissolved in the plasma.
When mature, the red blood cells of mammals do not have a nucleus (cell control center), which is why
they are called anucleate (meaning without a nucleus). This is the only mature cell among mammals that
does not contain a nucleus. Red blood cells do contain a nucleus early in their development, but push
them out as they develop to make room for more hemoglobin.
In other vertebrates (except for salamanders of the Batrachoseps family and fish of the Maurolicus family
[a ray-finned type of fish] with closely related species) red blood cells have a nucleus. Red blood cells
also lose other organelles so that they have enough room for hemoglobin. Organelle is a name for all of
the small bodies in the cell that perform necessary roles regarding the chemical reactions inside a cell.
Because of the lack of organelles, mature red blood cells do not contain DNA (deoxyribonucleic acid) and
cannot produce their own RNA (ribonucleic acid) or proteins. DNA is a chain of many connected genes.
Genes contain coded instructions for how proteins should be constructed and how certain bodily
characteristics should develop. RNA is a chemical substance that is important in building up proteins.
Because of the lack of DNA and RNA, red blood cells have limited ability to repair themselves. Because
viruses contain DNA and RNA genes, the lack of DNA and RNA in red blood cells means that viruses will
not evolve to attack red blood cells.
In humans and most mammals, red blood cells are round, flat, and concave on both sides (like a shallow
bowl) which increases the surface area for gas exchange across its membrane. They are flattened and
pushed in at the center, have a circular shaped cross section, and a ring-shaped edge. The shape of red
blood cells improves the properties of blood flow in large blood vessels such as maximizing the flow of
blood in parallel layers. The shape also decreases the thickening of artery walls by helping to regulate
platelets (cells that help change the blood from a liquid to solid form). Red blood cells are capable of
assuming a cigar shape which helps them maximize their surface and efficiently release the oxygen they
are carrying.
Even-toed hoofed animals (known as Artiodactyla) are an exception to the typical red blood cell shape
because they can have red blood cells of a wide variety of unusual shapes. For example, red blood cells
of camels and llamas are small and oval. Red blood cells of red deer/elk and mice are angular and
spherical, respectively. In large blood vessels, rouleaux formation can occur, which is a flat side by side
stack of red blood cells. This tends to occur if certain levels of protein are increased in the blood, which
can happen during inflammation.
In most mammals, the cell membrane (outer surface of the cell) of red blood cells contains half proteins
and half lipids (fats), which is what allows them to perform its functions such as changing shape (because
they are flexible), maintaining membrane fluidity (the membrane is a fluid structure), and resisting rupture
when travelling through the blood, especially the network of capillaries. In fact, red blood cells can
squeeze through capillaries that are less than half their diameter and when they emerge from the other
end they regain their original shape (much like a rubber band regains its original shape after it is no longer
being stretched).
There are more than 50 proteins in the cell membrane of red blood cells. About half of these proteins carry
the different blood group antigens (e.g., A, B, Rh, etc). These antigens are naturally made by the body and
the presence of them defines one’s blood type. Proteins in the cell membrane help move ions (electrically
charged particles) and other molecules across the red blood cell membrane. Proteins in the cell membrane
also help red blood cells attach to other cells and provide a response for the immune system. Proteins in
the cell membrane also help adhere to and interact with endothelial cells and signaling receptors.
Endothelial cells are cells that line the vessels of the body. Signaling receptors are receptors on cells that
(when stimulated) allow cells to cause responses in other cells.
In general, protein cell membranes are divided into groups depending on whether they help with cell
adhesion, transport, or cell structure, but some perform more than one function. The main cell adhesion
proteins in the cell membrane are known as ICAM-4 (intercellular adhesion molecule-4) and BCAM (basal
cell adhesion molecule). ICAM-4 binds to integrins which are cell surface receptors that mediate the
attachment of a cell to its surroundings. BCAM gives rise to a human blood group system known as the
Lutheran blood group system.
In terms of transport proteins in the cell membrane, there are some that pump sodium out of cells and
pump potassium into cells. This protein is known as NA+/K+ -ATPase (Na stands for sodium and K stands
for potassium). There is also a protein known as Na-K-Cl cotransporter which helps transport sodium,
potassium, and chloride in and out of cells (Cl stands for chloride). There is also the Na+Cl- transporter,
which reabsorbs sodium and chloride ions into cells. There is also calcium ATPase which transfers
calcium after muscle contractions. Sodium hydrogen antiporter is another protein that is responsible for
maintaining the sodium balance.
There is also a protein known as chloride potassium symporter which transports chloride across the cell
membrane. A protein known as the Gardos channel is responsible for potassium moving out of red blood
cells. There is Rh-associated glycoprotein, which appears to transport carbon dioxide. The Kidd antigen
protein transports urea (a chemical compounds found in urine). Another protein known as GLUT1 (glucose
transporter 1) helps transport glucose (a type of sugar) and L-dehydroascorbic acid (a form of vitamin C)
across the cell membrane. The protein Aquaporin 1 helps transport water across the cell membrane. There
is also Band3 which transports anions (particles with a negative electric charge) across the cell
membrane.
Cell membrane proteins that play a role in cell structure connect with proteins that make up the skeleton of
the cell, which prevents it from collapsing. They may also connect the lipid bilayer with the skeleton of the
cell. There are several such proteins that perform a structural role. One is Band3, which is also a
transport protein. Band3 composes 25% of the red blood cell membrane surface. Each red blood cell has
about one million copies of Band3. Band3 also helps make metabolons, which are temporary structures
composed on enzymes (such as enzymes that break down glucose or convert carbon dioxide and water)
and structural elements of the cell. Metabolons may play a role transporting gases and ions and may play
a role in red blood cell metabolism. Metabolism is a term for the chemical actions in cells that release
energy from nutrients or that use energy to create other substances.
A protein that is a major part of the cell skeleton is protein 4.1. Another is called dematin. Another group of
proteins that make up the cell skeleton are known as adducins. A protein known as glycophorin C and D
plays an important role in maintaining the shape of the red blood cell and the properties of the cell
membrane. A protein known as XK helps make the Kx antigen, which is responsible for determining a
person’s blood type. The RhD and RhCE proteins help define the Rh blood group, which is one of 30
human blood groups. The Duffy protein is a receptor on red blood cells for chemokines which are small
signaling proteins secreted by cells.
Many membrane proteins also interact with the lipid portion of the membrane. The lipid portion of the
membrane is mostly made of cholesterol and phospholipids (a special class of fat), divided into equal
portions by weight. Cholesterol is a waxy, fatty substance found only in animal tissues. It is evenly
distributed between the inner and outer leaflets (layers) of the cell membrane. There are five main types of
phospholipids. Certain proteins and patches of lipids can group together and form island-like lipid rafts.
Lipid rafts in red blood cells can mediate signaling of the beta 2-adrenergic receptor, which helps stimulate
fight or slight responses. Lipid rafts also increase cAMP (cyclic adenosine monophosphate) levels. CAMP
is a messenger in the body that helps transfer certain substances into cells which normally could not get in
without them. In other words, they are like an escort. cAMP allows for malaria parasites, for example, to
get inside red blood cells and reside there for part of its life while feeding on its hemoglobin. This breaks
the red blood cells apart and causes fevers.
Phospholipids are distributed unevenly between the inner and outer layers due to the function of
phospholipd transport proteins. Specifically, protein transporters known as Flippases move phospholipids
from the outer layer to the inner cell membrane layer. Other protein transporters known as Floppases
move phospholipids from the inner layer to the outer cell membrane layer. Scramblases are protein
transporters that move phospholipids in both directions at the same time. Some phospholipid transport
proteins require energy input in the form of adenosine triphosphate (ATP, which is a molecule that stores
energy) because they are moving against resistance. These transport proteins are referred to as energy
dependent. Some phospholipid transport proteins do not move against areas of resistance and thus do not
need ATP energy input. These transport proteins are referred to as energy independent.
Technically, there are three layers to the red blood cell membrane. The outer layer is made mostly of
carbohydrates and is known as glycocalyx. There are also two layers of lipid molecules which also contain
transmembrane proteins. These types of proteins go from one side of the membrane through to the other
side of the membrane. There is a structural network of proteins, known as the membrane skeleton, located
on the inside surface of the two layers of lipid molecules. Specifically, the outer layer is made of
phosphatidylcholine (PC) and sphingomyelin (SM). The other three phospholipids which make up the inner
layer are made of phosphatidylethanolamine (PE), phosphatidylserine (PS), and small amounts of
phosphoinositol (PI). There are more minor phospholipid components of the cell membrane such as
phosphatidylinositol 4,5-bisphosphate (PIP2). PIP2 and PS help regulate the mechanical function of the
cell membrane by interacting with cell membrane skeletal (structural) proteins such as spectrin and protein
4.1R. For example, cell membrane stability is improved when PS binds to spectrin. PIP2 helps bind protein
4.1R together with glycophorin C, which is a protein that helps maintain the shape of red blood cells and
the cell membrane properties. PIP2 decreases the interaction of protein 4.1R with protein band 3 (a type
of transport protein). By modulating these interactions, PIP2 may help link the cell membrane layers to
the cell membrane skeleton.
The uneven distribution of phospholipids in the cell membrane is important for the cell structure and
function for many reasons. First, it is important for PS to be part of the inner layer because when red
blood cells expose PS on the outer surface, they can recognized by macrophages and destroyed by them.
It can also cause red blood cells to stick to vascular endothelial cells, which are red blood cells that line
the entire circulatory system. When this happens, red blood cells cannot move normally through the blood
vessels.
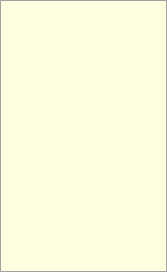
WHAT ELSE DO RED BLOOD CELLS DO?
When red blood cells undergo pressurized stress inside a blood vessel that is narrowing, they release
(ATP; see prior section) which causes the blood vessel walls to relax and widen, which improves blood
flow. When hemoglobin releases oxygen, red blood cells release thionitrites (types of organic structures
containing carbon) that also widens blood vessels to direct more blood to areas of the body that need
oxygen. Red blood cells can also make hydrogen sulfide, a gas that sends signals to relax the cell wall.
This is how garlic is believed to protect the heart - by taking sulfur compounds from the garlic and
converting it to hydrogen sulfide. This relaxes the cell wall, which protects the heart.
When red blood cells undergo pressurized stress, this can also activate them to use enzymes to make
nitric oxide (a type of molecule that transmits information between cells) which can contribute to the
regulation of the tone of blood vessels. An enzyme is a type of protein that helps produce chemical
reactions in the body. Specifically, red blood cells use enzymes called nitric oxide synthase and combine
them with oxygen, L-arginine (a type of amino acid), and a type of co-enzyme called NADPH
(nicotinamide adenine dinucleotide phosphate) to create nitric oxide. Amino acids are a group of
chemical substances that form proteins. A co-enzyme is a non-protein chemical compound that is bound
to a protein and is needed for the protein’s functioning.
Like red blood cells, white blood cells play a role in the immune system although nowhere near to the
same degree. Specifically, when red blood cells are broken down by invading substances (e.g., bacteria),
the hemoglobin releases free radicals, which kills it by breaking down the invading substance’s cell wall
and cell membrane. Free radicals are molecules with unpaired electrons (negatively charged particle that
is smaller than an atom). In their attempt to find another electron, free radicals are very reactive and
cause damage to surrounding molecules.
DO RED BLOOD CELLS USE THEIR OWN OXYGEN?
No. Red blood cells do not use the oxygen they transport because they do not have mitochondria.
Mitochondria are rod-shaped bodies that break down simple substances to provide energy. Red blood
cells instead get their energy by producing ATP (see earlier) after using the energy from converting
glucose (a type of sugar) into pyruvate (a type of acid).
WHY ARE RED BLOOD CELLS RED?
The heme group of hemoglobin is the reason that blood is red. Heme is the iron component of hemoglobin.
The blood is scarlet red when hemoglobin combines with oxygen, is a dark burgundy red color (and bluish
around the blood vessel wall and skin) when oxygen has been released, and is bluish when oxygen
deficiency is prolonged. When hemoglobin combines with oxygen it is known as oxyhemoglobin. When
oxygen it is released, it is known as deoxyhemoglobin. A device called a pulse oximeter (which fits over
one of the fingers like a clothespin) analyzes the color of the blood and uses this information to determine
how much oxygen is in the blood. Each human red blood cell contains about 270 million hemoglobin
molecules, each containing 4 heme groups.
HOW MANY RED BLOOD CELLS DO PEOPLE HAVE?
The average human male has between 20 to 30 trillion red blood cells, which is about 25% of the cells in
the body. This amounts to about 5 to 6 million (usually 5.2 million) red blood cells per microliter (one
millionth of a liter) of blood for men. Women have slightly fewer red blood cells (between 4 to 5 million per
microliter of blood; usually 4.6 million) than men. Each drop of blood contains millions of red blood cells.
There are about one billion red blood cells in two to three drops of blood. Red blood cells are much more
common than other blood particles such as white blood cells (about 4,000 to 11,000 microliters of blood).
For every 600 red blood cells, there is about one white blood cell and 40 platelets.
ARE THERE ANY ANIMALS WITH BACKBONES THAT DO NOT HAVE RED BLOOD CELLS?
Yes. Crocodile icefish are the only animals with backbones that are known to usually not contain red
blood cells. Their blood is yellow as a result because there is no hemoglobin (although remnants of
hemoglobin can be found) and because the blood plasma is yellow. Crocodile icefishes rarely have red
blood cells and when they do, they do not work properly. They get oxygen from living in oxygen-rich cold
water which transports oxygen that is freely dissolved through the blood. Compared to animals like these,
red blood cells helped vertebrates obtain higher oxygen concentrations in their bodies, less thick blood,
and better diffusion of oxygen from blood to tissues.
WHERE ARE RED BLOOD CELLS MADE?
Red blood cells are made in the bone marrow (a type of tissue inside of the bones) from stem cells (cells
that give rise to other cells). These stem cells are known as hematopoietic stem cells (also known as
hemocytoblasts). The process of going from stem cells to mature red blood cells is known as
erythropoiesis. This takes several days and is influenced by substances in the body known as growth
factors. The earliest form of a red blood cell is known as a pro-erythroblast. This eventually becomes an
erythroblast (also known as a normoblast) and then turns into an immature red blood cell known as a
reticulocyte. Reticulocytes make up about 1% of red blood cells circulating in the body. Reticulocytes
expel the nucleus but may still contain part of the endoplasmic reticulum, which looks like a fine netting.
The endoplastic reticulum is a complex system of folded, flat sacs that provide a large area for fluid to be
stored and for reactions to occur. Reticulocytes continue to make hemoglobin even without the cell
nucleus because the instructions from the nucleus are still in the cytoplasm. Once enough hemoglobin is
made, reticulocytes exit the bone marrow, lose the residual endoplastic reticulum, and become red blood
cells. About 2.4 million new red blood cells are produced per second in a healthy human body. In an
embryo, the liver is the main area where red blood cells are produced. An embryo is a fertilized egg from
the time of conception until the 8th week of pregnancy.
The process of making red blood cells is known as erythropoiesis and it occurs continuously in the bone
marrow of large bones. This process can be stimulated and quickened by a hormone known as EPO
(erythropoietin) which is made by the kidneys (90% of it is made there) and the liver. Hormones are
natural chemicals produced by the body and released into the blood that have a specific effect on tissues
in the body. The body will stop making EPO as long as oxygen levels remain normal. Red blood cells are
continuously moved by the pushing force of arteries and pulling force of veins as they squeeze through
tiny blood vessels.
In humans, some red blood cells are stored in the spleen. Other animals are able to use the red blood cell
storage capabilities of the spleen better than humans, such as horses and dogs. Specifically, these
animals can store a large amount of red blood cells which are later released into the blood during times of
exertion stress, allowing them to carry more oxygen to the body.
HOW LONG DO RED BLOOD CELLS LIVE?
Red blood cells circulate in the blood for between 100 to 120 days in the body (but 80-90 days in a full
term infant). At the end of their lives, red blood cells become unable to function properly due to old age,
rupture, and are removed from the blood stream by macrophages in the process of continuously purging
the blood. Macrophages are a type of white blood cell that eats bacteria and other foreign substances.
Macrophages remove red blood cells in the bone marrow, liver, and spleen. In a red blood cells full life-
span, they travel around the entire body about 75,000 times.
As they age, red blood cells shrink, become more rigid, stick to the walls of blood vessels, have
difficulties getting through the capillaries, and their cell membranes undergo changes (e.g., shrinkage,
irregular bulging, scrambling of lipid groups within the membrane) which allows it to be recognized by
macrophages and destroyed by them. Another change red blood cells undergo when they die is that a
component of the cell membrane known as phosphatidylserine is moved from the inside of the cell
membrane to the outside of the cell membrane. The process of removing red blood cells from the blood
stream is known as eryptosis and usually occurs at the same rate as red blood cell production.
Hemoglobin decomposes into iron (which can be reused by the marrow) and a greenish pigment known as
biliverdin. Some of the biliverdin is converted by the liver to bilirubin. Bilirubin is a yellow-orange
substance found in bile. Bile is a bitter, yellow-green substance released from the liver that carries away
waste products.
WHAT CAN CAUSE THE RED BLOOD CELL COUNT TO BE TOO LOW?
When too many red blood cells die, there will be an abnormally low number of them present in the blood.
The general term for this is anemia although anemia can also refer to too little hemoglobin in the blood,
which in turn prevents them from transporting enough oxygen to the body. When the bone marrow cannot
produce only red blood cells this is known as pure red cell aplasia. In aplastic anemia, the bone marrow
and stem cells are damaged, which causes a decrease in red blood cells, white blood cells, or platelets.
There are many other factors that contribute to the process of red blood cell death including decreased
energy, the activity of substances produced within the body, increased body temperature, stress to the
body caused by environmental pressures, stress from the rapid movement of water across the cell
membrane, increased movement of calcium ions [electrically charged particle] inside cells, increases in
ceramides (a family of waxy lipid molecules), and xenobiotics. Xenobiotics are chemicals found in the
body that are not normally produced in it or expected to be there.
There are many medical conditions that contribute to red blood cell death. The most common is iron
deficiency, which can be due to problems absorbing iron or not consuming enough food or drink in the
diet that contains iron. This prevents the formation of hemoglobin because hemoglobin contains iron.
Other medical conditions that can cause red blood cell death are deficiency of phosphate (a type of
salt), infection with mycoplasma (a type of bacteria that attacks the cell wall), malaria, kidney failure
(due to a lack of EPO), and diabetes mellitus. Malaria is a serious disease caused by parasites that is
spread by mosquitoes. In diabetes mellitus, the body is not able to effectively use a natural chemical
called insulin, which quickly absorbs glucose (a type of sugar) from the blood into cells for their energy
needs and into the fat and liver cells for storage. Sepsis can also cause the death of red blood cells.
Sepsis is a possibly deadly medical condition characterized by inflammation of the body due to severe
infection.
Other causes of red blood cell death include sickle cell anemia, inherited spherocytosis, elliptocytosis,
stomatocytosis, myelodysplastic syndrome, Wilson’s syndrome, and G6PD deficiency. Sickle cell
anemia is an inherited disease in which the red blood cells become sickle shaped due to abnormal
hemoglobin molecules releasing their oxygen load in the tissues. Because sickle-shaped red blood cells
are less elastic, they can cause medical problems such as blockage or rupture of blood vessels, pain,
and other tissue damage. In spherocytosis, elliptocytosis, and stomatocytosis ( types of blood
disease), red blood cells are sphere shaped, elliptical, and mouth shaped (respectively) instead of
having their usual shape which causes them not to function properly. In these conditions, the proteins
of the red blood cell membranes are deficient. In spherocytrosis, the cell membrane is also small and
weak. These abnormal red blood cells get destroyed by the spleen. The spleen is an organ near the
stomach that helps fight infection and removes and destroys worn-out red blood cells.
Myelodysplastic syndrome is a broad term for diverse conditions that damage the hematopoietic stem
cells in bone marrow that make white blood cells. Wilson’s syndrome is a rare inherited disorder in
which copper accumulates slowly in the liver and is taken up by other parts of the body. G6PD
deficiency is a common inherited disorder characterized by a lack of the enzyme, glucose-6-phosphate-
dehydrogenase. An enzyme is a type of protein that helps produce chemical reactions in the body.
Normally, the G6PD enzyme produces chemicals that protect the red blood cells against the effects of
stress.
Other medical conditions that can contribute to red blood cell death are beta-thalassemias, which are a
group of inherited blood disorders caused by problems with hemoglobin production. Thalassesmias (and
sickle cell disease) are more common in countries where malaria is common because these
abnormalities provide some protection against malaria.
Another cause of red blood cell death is hemolytic-uremic syndrome, which is a death characterized by
anemia caused by the death of red blood cells, acute (sudden) kidney failure, and a low platelet count.
Yet another cause of red blood cell death is paroxysmal nocturnal hemoglobinuria, an inherited blood
disease characterized by anemia due to death of red blood cells in the blood, red urine, and the
formation of blood clots. In this condition, the proteins of the red blood cell membranes are deficient.
In hemolysis, there is a rupturing of red blood cells and its contents into the surrounding fluid. A
decrease of red blood cells caused by hemolysis is known as hemolytic anemia. After a blood
transfusion, if there is a blood mismatch then some of the donated red blood cells can be destroyed
under the direction of the hosts antibodies (part of the body’s defense system). This is known as a
hemolytic transfusion reaction.
There are also microangiopathies (diseases of small blood vessels) which can cause red blood cell
death and red blood cells to break into small pieces known as schistocytes. One example is
disseminated intravascular congestion, which is the formation of small blood clots inside the blood
vessels and throughout the body. Another example is thrombotic microangiopathy, which is blood clot
formation in the capillaries and arterioles (small blood vessel that connects arteries to blood vessels)
due to injury to the lining of the blood vessels. When these diseases occur, they create strands of
fibrin, which is a fibrous protein involved in blood clotting. These strands can sever red blood cells while
they try to move past a blood clot.
Red blood cell death is slowed by urea, erythropoietin (see last section), and nitric oxide (a colorless
gas produced in the body that is known to have effects on the blood vessels). Deficiency of vitamin
B12, iron, and folic acid (also known as vitamin B9 and folate) cause severe reductions in red blood
cells because these vitamins are needed for DNA to synthesize all cells, especially fast dividing cells
which red blood cells are.
In mice, red blood cell death is increased by mutations (changes) in a group of cell proteins known as
Annexin; enzymes known as Klotho , cGMP-dependent protein kinase type 1, and AMP-activated
protein kinase; and a type of protein known as adenomatous polyposis coli and anion exchanger 1.
Mice with sickle cell anemia and thalassemia are also known to be associated with red blood cell
death. Thalassemia is an inherited blood disease caused by weakening or destruction of red blood
cells. Red blood cell death is decreased in mice with mutations is an enzyme known as
phosphoinositide-dependent kinase 1 and janus kinase 3; protein receptors known as platelet-
activating factor receptor 6; a group of ion channels (ions are electrically charged particles) known as
transient receptor potential channels; and a transporter of the organic acid, taurine.
WHAT CAUSES THE RED BLOOD CELL COUNT TO BE TOO HIGH?
A high red blood cell count is usually defined as more than 5.72 (4.6 to 6.2) and 5.03 (4.2 to 5.4) million
red blood cells per microliter of blood for men and women, respectively. In children, what constitutes a
high red blood cell level depends on the age and gender.
Red blood cell counts increase at high altitudes because the body needs more oxygen. In fact, any
situation, behavior (e.g., smoking) or health condition that decreases the amount of oxygen in the body
(e.g., due to lung/heart disease or poor lung/heart function) increases the level of EPO in the body,
which stimulates red blood cell production as described earlier. If the kidneys release too much EPO,
this can also result in an increased red blood cell count. Artificially increasing EPO (EPO doping) to
cheat in athletic competitions will also increase the red blood cell count. Sometimes, the bone marrow
can malfunction, producing too many red blood cells. If the red blood cells cannot carry enough oxygen
(known as a hemoglobinopathy), more red blood cells might be produced to carry more oxygen. If there
is a loss of blood plasma (usually due to depletion of sodium and water), a high amount of red blood
cells can be produced.
Specific conditions that cause an abnormally high number of red blood cells are known as
polycythemias or erythrocytoses. In polycythemia vera, too many red blood cells are caused by an
abnormality in the bone marrow. Because there are too many red blood cells present, this makes the
blood thicker, which can cause various problems such as itching and blood clot formation. Other
conditions known to cause a high red blood cell counts are kidney cancer, dehydration, kidney
transplant, and use of anabolic steroids. Another cause of increased red blood cells is sleep apnea,
which is a disorder in which the person does not breathe for periods of time while sleeping. Another
cause is pulmonary fibrosis, which is the formation of scar tissue in the connective tissue of the lungs.
that are characterized by long-term or permanent narrowing of small airways (known as bronchi)
connected to the lungs. Poisoning with carbon dioxide (a type of gas) can also cause an increase in
red blood cells.
WHAT OTHER BLOOD TESTS INVOLVE RED BLOOD CELLS?
Besides the red blood cell count, there are several other blood tests involving red blood cells:
Erythrocyte sedimentation rate: The speed by which red blood cells settle to the bottom of a column of
blood in a glass tube.
Hematocrit: The percentage of the amount of blood that is occupied by red blood cells that are packed
together.
Hemoglobin: A red substance (made of iron and protein) in red blood cells that carries oxygen to the
cells in the body from the lungs.
in a given number of packed red blood cells.
There is also blood typing, in which determination of a person’s blood type is made from a blood sample
before a blood transfusion or organ transplant. Lastly, in a peripheral blood smear (blood film), a thin
layer of blood is smeared on a microscope slide and analyzed under a microscope to diagnose
diseases of the red blood cells.
WHAT HAPPENS TO RED BLOOD CELLS AFTER THEY DIE?
After red blood cells die and are broken down, the elements are recirculated throughout the body. The
heme part of hemoglobin is broken down into iron and bilverdin. Bilverdin is a green bile pigment. Bile is
a bitter, yellow-green substance released from the liver that carries away waste products. The bilverdin
is reduced to bilirubin. Bilirubin is a yellow-orange substance found in bile. When red blood cells are
broken down, bilirubin is released into the blood plasma and sent to the liver bound to albumin. Albumin
is the most abundant protein in the body. It is produced in the liver. Albumin binds to certain substances
(such as bilirubin) and helps retain them in the body so they are not all filtered out. The iron is released
into the plasma and is recirculated by a carrier protein known as transferrin (which binds to iron).
Almost all red blood cells are removed in the manner described here before they are old enough to
rupture. When red blood cells rupture, the hemoglobin binds to a protein called haptoglobin, which is not
excreted by the kidney.
HOW BIG ARE RED BLOOD CELLS?
Red blood cells are much smaller than most other human cells. They are about 6 to 8 micrometers in
size (diameter) with a thickness ranging from 0.8 to 2.5 micrometers. A micrometer is a very small unit
of length that measures one millionth of a meter. A meter is approximately 39 inches (slightly more than
3 feet). The size of red blood cells differs significantly, however, among animals with a backbone. The
red blood cells of mammals, are generally smaller than those of other animals with a backbone. On
average, the width of red blood cells are about 25% larger than the width of the capillaries. This has
been hypothesized to improve the transfer of oxygen from red blood cells to tissues.
HOW MUCH IRON IS IN RED BLOOD CELLS?
In the average adult human male, red blood cells contain a total of 2.5 grams of iron. This is about 65%
of the total amount of iron in the human body.
WHAT ARE PACKED RED BLOOD CELLS?
Packed red blood cells (pRBCs) are red blood cells that have been donated/collected, processed (e.g.,
removing white blood cells), and stored in a blood bank for use in a blood transfusion. Red blood cells
can be separated from the blood plasma in the laboratory with a centrifuge, which spins a blood sample
in a vial at very high speeds. This process is known as blood fractionation. In some cases, it is the
plasma that is donated and not the red blood cells. For this to happen, the red blood cells are
immediately pumped back to the donor and only the plasma is collected.
The blood is safe to donate and store because the body is always making more if it. Donating just the
red blood cells helps treat anemia without increasing blood volume. After donating blood, people
sometimes feel lightheaded because of the blood of oxygen from the red blood cells and because of the
loss of blood sugar that provides energy. However, this side effect is only temporary.
People who benefit most from donated red blood cells are people who suffer acute bleeding in
emergency situations such as trauma. People suffering from chronic anemia such as patients with
kidney failure or gastrointestinal bleeding also benefit significantly from red blood cell transfusions. It is
important to remove white blood cells by filtering them out shortly after they are donated before storing
red blood cells. This is because if there are high numbers of white blood cells left in the donated
sample, they can break apart, deteroriate, and release cytokines. Cytokines are proteins that help
other white blood cells (and other cells) communicate with each other and can contribute to
inflammation. It is because the white blood cells can release cytokines that they have been implicated
in causing reactions to blood transfusions by the recipient.
HOW ARE RED BLOOD CELLS USED IN BLOOD DOPING?
In blood doping, athletes try to cheat by altering their blood to provide them with more energy. Red
blood cells are ideal for this because they provide the person with more oxygen. The process is done
by removing a liter of blood and then isolating, freezing, and storing the red blood cells. The red blood
cells are then injected into the body shortly before the athletic activity. Some people inject themselves
with erythropoietin, a hormone that increases red blood cell production.
Because athletes are injecting themselves with their own red blood cells (or a natural substance that
increases red blood cells), it can be difficult to detect this form of cheating. However, a side effect of
increasing the number of red blood cells in the body is that it increases the thickness of the blood. The
heart and blood vessels are not capable of handling this and damage to these structures can occur as
a result.
HOW LONG CAN RED BLOOD CELLS BE STORED FOR?
Red blood cells can generally be stored for up to five weeks at -79 Celsius although some report
storage for up to 6 weeks. If they are treated and frozen, they can be stored for 10 years or more.
CAN RED BLOOD CELLS BE GROWN ARTIFICIALLY?
Yes. This was announced as having been done in 2008 in which human embryonic stem cells were
transformed into red blood cells after exposing them to a sequence of nutrients and growth factors
(natural substances which can stimulate cell growth). This turned them into hemangioblasts or
erythroblasts, which are precursors to red blood cells. Erythroblasts can create millions of hemoglobin
molecules per second. From there, they turned into red blood cells. Artificial red blood cells may be
used for blood transfusions in the future. As noted earlier, red blood cells do not have a nucleus.
Getting cells to eject their nucleus was difficult but was accomplished by growing red blood cells on
stromal cells (connective tissue cells) from the bone marrow (where red blood cells are normally made).
By growing them where red blood cells are normally made, this influenced expelling of the nucleus.
WHAT IS THE ZETA POTENTIAL OF RED BLOOD CELLS?
The zeta potential is the degree of negative electric charge on the surface of a red blood cell, which is
expressed in millivolts (one thousandth of a volt). The normal zeta potential of red blood cells is -15.7
millivolts. This is largely due to sialic acid residues in the membrane of red blood cells that get exposed
because when sialic acid is removed from red blood cells, the zeta potential changes to -6.06 millivolts.
Sialic acid is an acidic substance that attaches to the surfaces of cells and certain proteins.
WHEN WERE RED BLOOD CELLS DISCOVERED?
Red blood cells were first observed and described in 1658, by the Dutch biologist, Jan Swammerdam,
when he used a microscope to study the blood of a frog. In 1674, another Dutch scientist, Antonie
Philips van Leeuwenhoek, independently described red blood cells after also studying them under the
microscope. He provided a more thorough description and estimated their size to be 25,000 times
smaller than a grain of sand.
WHAT ARE THE OLDEST RED BLOOD CELLS EVER FOUND?
The oldest red blood cells ever discovered were found in Otzi the Iceman, a well-preserved mummy of a
man who lived in about 3,300 BC. In 2012, scientists announced that he still had intact red blood cells.
His red blood cells were the same size and appearance as those found in modern times.
WHAT ELSE ARE RED BLOOD CELLS KNOWN AS?
Red blood cells are also known as erythrocytes, red cells, haematids, and erythroid cells.